
All You Need To Know About Wireworm
- Scientific opinion
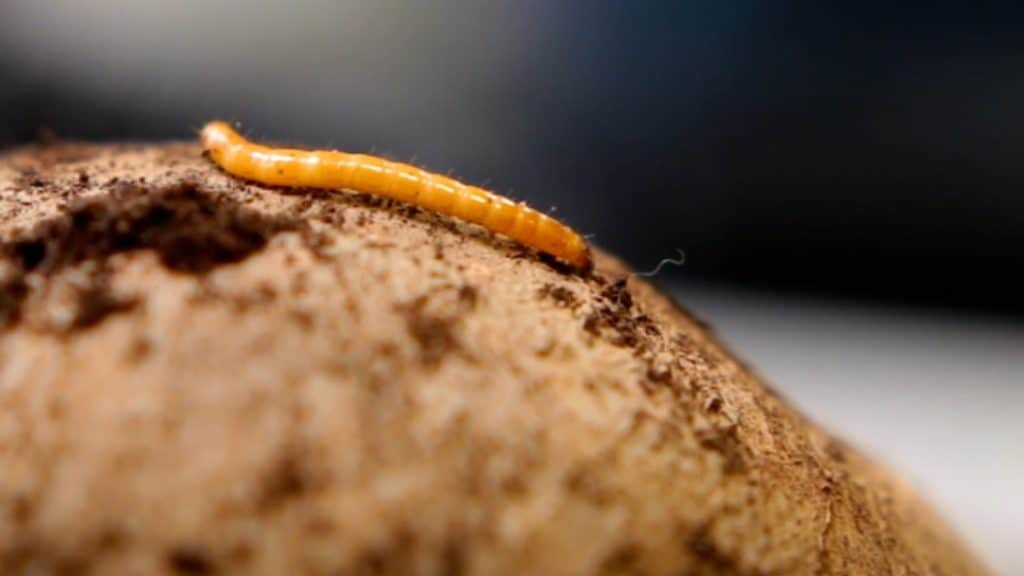
Wireworm: the basics
The damage to agricultural produce caused by wireworm has increased massively in recent years. There are a number of reasons for this, including the loss of effective insecticides, changes in cultivation practices, such as the min-till practices encouraged by regenerative agricultural regimes, and climate change. We desperately need new ways to combat wireworm and need to consider holistic integrative pest management strategies.
Wireworms are the larvae of click beetles and are small, soil-dwelling pests of agricultural land. The three main click beetle species that are responsible for the most damage to crops, like potatoes, in the UK are Agriotes obscurus, lineatus, and sputator. Adult click beetles lay their eggs just beneath the soil surface and it takes 4 to 6 weeks for the wireworms to hatch.
Optimal conditions for wireworms are found in weedy grasslands, because the soil is undisturbed for years and this preserves their natural habitat. But wireworm can also be found on arable land. Once they have hatched, the wireworm can live in the soil for 4-5 years and they need live plant material as a food source to survive. When this plant material is a farmer’s crop then this is the beginnings of major problems. For instance, wireworms cause potatoes to become unmarketable due to them leaving small narrow holes and scarring across the periderm.
Detection and control of wireworm are going to be increasingly important in coming years as chemical controls are withdrawn from the market for environmental reasons. We need to understand the enemy and this blog article gathers our knowledge of wireworm biology, their distribution, how we detect them and what we can then do about it.
Cutting open potatoes can reveal the extent of wireworm damage on the side of the flesh.
So what are wireworm?
There are more than 9,000 species of click beetles distributed worldwide, and about 60 of these are found in the UK. The larvae of the click beetles are given the name wireworms, because they have a wire-like appearance, but they look rather different from the traditional earth worms. Only a few wireworm species are actually agricultural pests in the UK, and three key species (Agriotes lineatus, A. obscurus, A. sputator) are responsible for most of the damage to potatoes in the UK [Parker and Howard, 2001]. A forth species, A. sordidus, has also been found to cause damage to crops in the UK, but it is much less common and has only been recorded in warm, coastal places such as Essex to South Devon [UK beetles, 2021].
Rather than having a single food source, wireworms are generalist feeders and like to eat the roots, shoots, and bulbs of various crops, such as potatoes, carrots, maize etc. The main effects of wireworm feeding on neck and belowground plant organs are seedling mortality, and reduced nutrient and water supply if tunnels are bored through the roots and lower stems [Ritter and Richter, 2013].
Why are wireworms such a problem in the UK potato industry?
Potato crops are particularly susceptible to wireworm attack because the damage to tubers reduces crop quality rather than yield [Parker and Howard, 2001]. Damage usually appears as small holes , and narrow tunnels that are a few millimeters deep, and scarring to the periderm [Barsics et al, 2013].
Wireworms crawl over the surface of a potato and burrow into the flesh.
A 2021 survey of UK potato farmers showed that they regarded wireworm-related damage, along with potato cyst nematode as the number one factor causing crop to not reach its target value. Even small levels of damage can lead to a whole field of potatoes losing >90% of their value and this is a massive risk for potato farmers. As a result, potato farming in the UK is declining and in recent years more than 100 UK potato growers have stopped farming potatoes due to increasing risks outweighing the rewards (AHDB, 2021). The potato industry is currently worth around £2.5Bn directly to the UK economy and needs to be safeguarded from wireworm -this requires that we understand as much as we can about the adult insects and the larvae.
Potato crops that are planted in fields after long-term grass growth (four years or more) have always been considered to be at the greatest risk of wireworm attack, since wireworms favour undisturbed soil. Around 60-70% of long-term grass fields are infested with wireworm. However, wireworm damage has increasingly been seen in arable-only rotations, and this upward trend may continue as reduced tillage regimes become more popular between potato crops.
Once a field is infested, wireworms are difficult to control because of their long-life cycles, overlapping generations, their adaption to a wide range of ecosystems (e.g., arable land and grasslands), and their ability to react quickly to abiotic changes by migrating down the soil profile [Rogge et al, 2017]. This efficacy makes short term management strategies ineffective. If we layer on top of this recent withdrawal of insecticides (e.g., Mocap in 2019) because of environmental and human health concerns, and climate change increasing UK temperatures (larvae prefer warmer soils) [Kuhar and Alvarez, 2008] then we have a serious problem. But wireworms are not just a problem for the UK potato industry!
What about wireworm in other countries and other crops?
Across the world, wireworm can cause damage to an extremely wide range of crops, such as grain (i.e., wheat, barley, oats), forage (corn, maize), tobacco, most vegetables, sugar beets, sugar cane, sweet potatoes, and small fruits (e.g., strawberries). This also causes major losses in agricultural produce. For instance, crop losses in North America due to wireworm damage range from 5-25% [Utah State University, 2020], in France attacks on just 10 % of maize plants in a field correspond to a loss of 0.5-1 tonne per hectare whilst on Prince Edward Island, Canada, wireworm damage cost the potato industry over $10 million in 2018 [CBC, 2021].
In small grains, wireworm damage can reach 65% on standing crops, which may require replanting (Smith et al. 1981). Wireworms are a serious pest in corn, particularly in America, causing up to 35% crop damage per field [FAO, 2005; Apablaza et al. 1977]. They feed on the germ of corn kernels or completely hollow out the seeds, leaving only the seed coat, and this damage results in gaps in the rows.
Wireworms may also cut off small roots or tunnel into the underground portions of the root or stem of young corn plants and the plants appear stunted or wilted [Purdue University, 2009]. However, in North America Agriotes spp. is not considered to be a major pest like in Europe, and there are other wireworm genera that are more damaging including Limonius spp., Selatosomus spp., Conoderus spp., Melanotus spp., and Dalopius spp. [Nikoukar and Rashed, 2022].
Maize is also susceptible to damage by wireworm attack – the worms eat small roots and can significantly reduce yields.
What insights can we get from click beetle and wireworm biology, including seasonality?
Click beetle biology
Click beetles get their name from the clicking noise made by the flexible union of the prothorax and mesothorax that the beetles can flex and snap [Yonuis et al, 2015]. We think that the motion and the clicking noise are used to partly to ward off predators and partly for the beetle to right itself if it is on its back [Triplehorn and Johnson, 2005].
Adult click beetles can grow up to 15 to 33mm, are typically brown or black in colour [Rashed et al, 2015] and are nocturnal. The lifespan of the adult click beetle depends on species; some do not overwinter and lay their eggs a few days after mating, whilst others live for months and overwinter, laying eggs over a long period of time. The UK pest species A. obscurus, A. lineatus, and A. sputator are in this second category [Barsics et al, 2013].
An adult click beetle.
The seasonal activity of adult click beetles varies depending on species. For instance, the swarming period of A. lineatus is between May to the middle of August [Kozina et al, 2012], A. obscurus has been found to be active between May and June [Sufyan et al, 2014] and the adults of A. sputator are active from March/April, once the soil temperatures reach 10 degrees Celsius, until June.
Female click beetles tend to lay between 100 to 300 eggs singly or in clusters. The eggs are spherical (0.5mm diameter) and translucent; and are deposited just below the soil surface [Parker and Howard, 2001]. Females much prefer to lay eggs on grasslands rather than arable land since the eggs are better protected from desiccation [Barsics et al, 2013].
Eggs can take up to 4-6 weeks to hatch in the UK depending on the species and soil temperature, and when the wireworm hatch, they require live plant material to survive [Parker and Howard, 2001]. For A. obscurus, larval development can occur over four years and, without treatment, wireworm can damage crops for much of this development period. A. lineatus hatch within 2-4 weeks and the larvae immediately begin feeding on roots and tubers for up to four years. For A. sputator, larvae emerge after about 3 weeks, and development takes between two and four years depending on food and temperature.
Wireworm biology
Wireworms vary in size depending on the stage of their lives. Young larvae are usually white and around 1.5 mm long, but, depending on the species, they can grow up to 14 to 25 mm in length [Vernon and van Herk, 2013]. Agriotes sputator can get to 17mm in length whilst A. obscurus/lineatus are a somewhat larger and can grow up to 24mm [Falconer, 1944]. However even at their largest wireworms are only a couple of centimetres long and 1-2 mm wide, so can be extremely difficult to spot in soil.
Wireworms have a hard, shiny exoskeleton which makes them tougher than other pests/worms. They are orangey/brown (or toasted caramel) in colour with a narrow, segmented body. The wireworms have chewing mouthparts, and 3 pairs of short legs behind the head. They have clusters of sensilla on their labial and maxillary palps which help them locate host plants from the chemical cues that they give off, such as carbon dioxide [la Forgia et al, 2019]. This is important, since it suggests that chemical signals could be used in traps to attract wireworms, and it is on this principle that larvae traps have been produced and used.
The periods during which wireworms are active depend on the species and the country they are found in. In the UK, wireworms usually have two intense activity periods that can result in significant crop damage, depending on the temperature and moisture conditions in the different soil layers [Poggi et al, 2021]. They are active from March to May and from September to October [Balachowsky and Mensil, 1936]. Climate and soil properties influence the vertical migration of wireworms through the soil profile and therefore affect the levels of damage done to crops [Poggi et al, 2021]. For example, A. obscurus seems to have periods of reduced activity when the soil is warmest and driest, and wireworms may move further down into the soil to avoid this [Falconer 1944].
With regards to the main pests found in the UK, A.obscurus has been observed to start damaging crops during their first spring (a year after eggs have hatched), and they become increasingly more harmful the older they get. A. lineatus begins doing noticeable damage at around 2 years old when it has reached a damageable size (10mm) [Barsics et al, 2013].
Schematic depiction of the life cycle of a click beetles and the wireworm larval stages.
Where do you find wireworms and click beetles?
The most likely place to find wireworm is in grasslands, where the continuous grass growth provides a suitable habitat due to the abundance of food, stable moisture & temperature conditions, limited disturbance, and vegetation cover [Saussure et al, 2015]. Arable fields that were once grasslands can still be highly infested with wireworms, particularly when you consider the 4-5 year lifespan of a wireworm. Estimates of wireworm prevalence in these kind of fields vary, but some researchers have found that fields that have been grassland for 10 years or more have a one in two chance of being infested, and this increases to a three in four chance for permanent pasture [Parker and Seeney, 1997]. They have also been observed to be more abundant in weedy fields than in weed free fields, and so keeping weeds at a low level might reduce infestation.
How does soil affect wireworm levels
Soil characteristics such as pH, moisture, and temperature, along with rainfall and plant species are dominant factors influencing the spatial distribution and abundance of wireworms in an area [Milosavljevic et al, 2016]. The levels of organic-matter is also believed to be a strong risk factor for economic crop damage [Furlan et al. 2016] – this may be because soils rich in organic matter are also likely to have more weeds and a higher moisture content, which could result in a higher survival rate for young larvae.
Wireworm tend to avoid dry soil and will die if they stay too long in it, but they can also suffocate and die if they stay in water saturated soil [Campbell 1937]. Extreme temperatures of under 2°C and over 30°C, respectively, can cause death. Optimum soil temperatures were found to be around 10 to 25 degrees Celsius, and wireworms can be found at a depth of 8-10cm in the soil when those conditions are met.
Soil bulk density is lower on average in fields with high wireworm infestations, which can be seen as an indication of soil structure rather than soil mineral content. Soils which are less dense are potentially easier for wireworms to move through and are less prone to becoming waterlogged [Parker and Seeney, 1997]. Soils with a higher clay content may be more ideal at sustaining a wireworm population, even though larvae can move more easily in sandy soils, and this may be because soils with a higher clay content can retain a higher humidity for longer, and thus lead to lower egg and larval mortality in the first phases of the wireworm life cycle [Lefko et al, 1998; Furlan et al, 2016]. For most wireworm species the most favourable soil moisture content is between 9 and 12%.
In the UK, A. obscurus and A. lineatus tend to occur in higher altitude areas, which are characterised by lower temperatures, higher precipitation, and acidic, humous soils. A. sputator can be found in similar areas to A. obscurus/lineatus, but also in locations with a warmer, drier climate and alkaline soils due to its euryoecious nature [Ritter and Richter, 2013].
Where is the preferred habitat of adult click beetle and how do they move around?
There is actually relatively little concrete evidence on the spatial distribution of adult click beetles, but the investigations that have been carried out used pheromone traps to track the beetles. There seems to be a lot of variation between close together sites [Blackshaw and Vernon, 2006] suggesting that both adult male A. lineatus and A. obscurus distributions tend to cluster together at the landscape scale, and that there are significant associations between the two species, but there are significant gaps in our knowledge on this topic.
Permanently grass-covered land, such as pastures, represents a perfect habitat for wireworm. Surrounding hedgerows and weed-covered land are particular risk factors and seem to attract click beetles
Over a short range, adult beetles seem to walk to get around [Roebuck et al, 1947; van Herk and Vernon 2020] and field studies suggest that, most of the time, adult male and female Agriotes make rather short journeys around the places that they pupate and emerge. However, large-scale, directional movement can also occur [Roebuck et al, 1947; Sufyan et al, 2007] and studies in Canada suggest that flight occurs in most species [Boiteau et al, 2000]. The three UK Agriotes species have been observed in flight, mainly in moist, humid conditions both in daylight and after sunset, but flight activity varies between individuals [Brian, 1947].
As might be expected from the reduced mobility of click beetles, wireworm damage is usually patchy which suggests that they are distributed into “clumps”. The distribution of Agriotes wireworm can look random, with some study plots being heavily infested while others close are rather less so [Salt and Hollick 1946]. As wireworms age, so their spatial distribution increases, suggesting that they do gradually move over time. But, on the whole Agriotes larvae rarely disperse between crops or move too far in the soil (though they will travel vertically up and down the soil profile), especially if local food supply is sufficient [Schallart et al, 2011]. There is evidence that wireworm can travel as far as 3.6 meters horizontally in 24 hours, an awfully long distance for a 1 cm long worm [CBC, 2021].
How do we detect and manage wireworm infestations in the field?
Currently, the best way that farmers can deal with wireworm problems is to carry out a basic risk assessments, by examining particular characteristics of the field, following this up with various trapping methods to understand the population levels. But there are then very limited controls to manage the populations. The detection methods and controls discussed below are similar across all countries with wireworm problems.
Wireworm risk assessments
Before a farmer plants a suspect field with a potentially vulnerable crop, it is always important to carry out risk assessments to assess the problem. A calendar of activities, based on years of accumulated knowledge, might be:
- Assess site characteristics (e.g., history of grassland, presence of grassy margins, rotation etc) around 12 months before potato crop.
- Deploy pheromone traps in the preceding year – May-June in previous crop.
- Take soil samples in October-March preceding planting.
- Undertake bait trapping assessments in February to March preceding planting.
Where a strong risk of damage is identified and a crop will still be planted, choosing an early maturing variety enables the farmer to lift his crop early to avoid peak wireworm activity in September or October. But where possible, most farmers are advised not to grow potatoes in a field with a wireworm infestation.
Several sampling methods for assessing infestation rate (number of wireworms per unit of surface) have been developed over time, such as bait traps, soil sampling, and pheromone traps, but these don’t really produce accurate information. The can help to refine or confirm risk assessments and produce numbers that can feed into control strategies, as discussed below.
Soil sampling
One of the most basic sampling strategies involves taking soil samples to assess wireworm population size, which can provide an estimation of subsequent damage in potato crops. It is best to sample soil in autumn and early spring, ahead of planting. The method was developed in the 1940s to sample wireworm, uses 20 10cm soil cores for a 4-10ha field and an aggregated sample is then analysed in the laboratory for larvae numbers. However, it is difficult to make this estimate accurate because populations can be patchy and only a few soil cores are typically taken to represent a whole field.
Soil sampling involves looking within a pre-defined volume of soil to count the number of wireworms present.
Traditional soil sampling is an expensive and labour-intensive process, and there is a poor correlation between sample results and damage seen in the crop. Additionally, even at the lower limit of detection in potato crops, which is equivalent to around 60,000 wireworm per hectare (AHDB, 2021), severe damage can still be caused.
Bait traps
Bait traps are small, perforated plastic containers part-filled with grain and buried in the soil for 10-14 days in late March to early April, when soil temperatures are higher than 10⁰C. The germinating seeds release carbon dioxide and it is though that this attracts wireworm into and around the trap. The traps are dug up and the wireworms counted.
If we compare the effectiveness of bait trapping and soil sampling, it seems that bait trapping is either as effective, or better, than soil sampling in detecting wireworms. Processing times for bait traps are also considerably less than for soil samples [Parker, 1994], but bait trapping tends to be less effective when alternative food sources are present, which is often the case in freshly ploughed grass fields.
An important question with all sampling is what the findings actually mean for risk assessments. According to researchers who tried to quantify risk a rough estimate is that an average of one wireworm per trap is equivalent to 20,000 wireworms per acre [Bechinski et al 1994]. When bait traps have an average of two to four wireworms, the risk is considered very high and trying to apply some kind of chemical control at planting is recommended. If the traps have more than four wireworms, the level is considered extreme, and the recommendation is not to plant potato in that field.
Pheromone traps
Pheromone traps incorporate chemical signals to attract adult click beetles, which are then trapped and can be counted. The most popular pheromone trap is the Vernon trap. It is a 15 × 15 × 5 cm high polyvinyl chloride box or plastic “pit fall” trap, dug slightly into the soil, with 2 ramps allowing beetles to walk, then fall into the interior holding area. The bubble cap pheromone lures are designed to be specific to the species that the farmer wants to catch, at least in theory [Van Herk et al, 2021].
Pheromone traps are placed in or around the edge of fields that will have potatoes planted in them the following spring. Pheromone traps are much less labour-intensive and much more sensitive than soil sampling, and trapping is best done in short periods (three to four days) in early May – leaving traps longer than this risks attracting beetles from outside the field.
Although pheromone traps can detect low levels of adults and provide an early warning of potential wireworm issues in the next crop, they don’t actually tell us anything about the wireworm that are already present in the field, and so the information should be backed up by other methods such as soil sampling or bait trapping.
A pheromone trap involves a cup-shaped section that is buried into the ground and a lid that incorporates the pheromone tube. A grid allows click beetles to crawl through, and they fall into the bottom of the trap.
Evolving methods
At B-hive innovations, we have recently undertaken a feasibility study to see whether we could detect wireworms automatically by computer vision approaches. Our approach involves mounting a camera to film soil after it has been turned up during ploughing and cultivating, and this approach will allow automated wireworm detection.
Our preliminary work suggests that we might have uncovered a new way of detecting wireworm in fields, but the methods still need optimisation before they can be rolled out to farmers – watch this space.
Other promising technological advances involve use of DNA barcoding to detect the presence, and species, of wireworms in fields [Staudacher]. The DNA can potentially be recovered from the environment and amplified in laboratories to give detectable amounts. If pieces of DNA that are specific to different click beetle species can be amplified and detected then this may be a powerful tool, and there is a potential for this approach to be used in conjunction with other soil sampling methodologies.
As agricultural machinery turns over soil it can bring wireworm to the surface allowing them to be detected by scientists visually, or by camera systems.
What control methods can be used to get rid of wireworms
Wireworms are really rather difficult to control due to them inhabiting the soil, the fact that they have a long-life cycle, and that our knowledge of their biology is incomplete. However, a recently-published, fully-assembled, gene sequence for Agriotes lineatus will help move research work forwards [King et al 2023].
If initial risk assessments and trapping suggests serious levels of wireworm activity in a field, control measures need to be implemented to manage the wireworms. The current best control measures rely on a mixture of chemical, biological, and cultural practices, along with knowledge on the wireworms species’ ecology to create a sustainable integrated pest management strategy.
Chemicals used to control wireworms
Using insecticides to treat wireworm infested fields is difficult for a number of reasons.
- Wireworms are located at varying depths in the soil profile and thus the insecticides need to be applied pre-planting to ensure they are incorporated into the soil correctly.
- Insecticides need to be used consistently throughout the season to cover the two wireworm feeding periods that extend across several months [Parker and Howard, 2001].
- Insecticides such as lindane and fipronil were effective at killing wireworms but have since been banned in the UK.
- There are fewer insecticides available on the market that can be used to treat wireworm effectively because many that were once used (e.g., aldrin which was used in the UK until 1989) contained organochlorines which are now banned due to environmental and health concerns [Grove et al, 2000].
In several lab and field studies it appears that different species respond to insecticides in different ways. For instance, A. sputator and A. obscurus were more susceptible to both clothianidin and chlorpyrifos than Limonius canus, but that the time needed for the Agriotes species to die after exposure was more than twice as long as required for the likes of L. canus, C. pruinina, and C. destructor [van Herk et al. 2007].
Another problem with chemicals such as neonicotinoid (e.g., clothianidin and acetamiprid), pyrethroid (e.g., tefluthrin) and spinosyn (i.e. spinosad), is that they have been noted to cause long-term morbidity in wireworms, from which they can then make a full recovery [Vernon et al, 2007].
In Canada the company BASF have recently created a new insecticide called Cimegra. Its active ingredient is broflanilide, and it has been found to be effective at killing wireworms by targeting their nervous system (BASF, 2021). Unfortunately, broflanilide has not been approved for use in the UK, and there are currently no other insecticides that are effective at killing wireworms available in the UK .
Using biological controls to combat wireworms
Biological controls involve using living organisms that are natural predators or parasites of wireworms, such as entomopathogenic fungi and nematodes. These species are introduced into the environment that is affected by wireworm, but whether or not biocontrols actually work is complex and can be affected by the likes of environmental conditions and wireworm density – they are certainly not reliable all of the time [Jaronski, 2010].
Beauveria and Metarhizium are genera of fungi that can reduce wireworm damage [Jansson and Seal, 1994] and the commercial strain of B. bassiana can reduce wireworm population in the field, compared to untreated control plots [Ladurner et al., 2008]. However, the mortality rates caused by B. bassiana under laboratory conditions were significantly higher at 50%, compared to field applications [Sufyan et al., 2017], which shows that the efficacy can drop significantly under real conditions.
High concentrations of M. brunneum conidia can cause significant wireworm mortality in pot experiments and the fungus can persist in the soil for up to 8 months [Rogge et al 2017]. However, this fungus also struggles in real world applications and in field trials only 2 out of the 10 field trials showed a reduction of potato damage that was statistically significant [Reinbacher, 2021]. Getting the conditions right for fungal formulations and applications may increase the effect on wireworms, but is going to take a large R&D effort, time and funding to perfect.
Entomopathogenic nematodes are parasites of insects and mainly belong to the two families of Steinernematidae and Heterorhabditidae [Burnell and Stock, 2000]. Like pathogenic fungi, which species of nematode to use is important factor since not all wireworm are susceptible to each nematode. For example, different strains of Heterorhabditis bacteriophora caused 17% to 67% mortality in the larvae of A. lineatus, whereas Steinernema feltiae did not infect this species at all (Ansari et al. 2009). Infection also depends on the developmental stage of the wireworm and environmental conditions [Lewis et al, 2006].
Cultural and mechanical controls
Currently, the simplest tools in a farmer’s armoury is to adopt a range of cultural practices, carried out before, during and after planting of crop seeds or seedlings, that involves modifying the soil environment. A big problem, though, is that some commonly recommended practices to improve soil conditions and to maximize profit, such as minimum/no-tillage, lack of proper rotation, and continuous cropping make field conditions favourable for wireworms, and this may contribute to increased wireworm populations [Adhikari and Reddy, 2017].
Ploughing the land can help to reduce wireworm numbers by exposing them to predators, like birds, when the soil is upturned, and by altering the structure of the soil, making it unsuitable for both wireworms and laying eggs in [Salt and Hollick, 1949]. Practices that are now associated with regenerative agriculture, such as minimum till or no till, mean that the soil remains undisturbed and an optimum environment for wireworms to live. Incorporating some ploughing as part of the standard rotation may represent the best of both worlds, but will require long-term monitoring to build evidence for the correct tilling approaches and frequencies.
As farmer’s plough their fields the soil is turned over bring wireworms to the surface. These wireworm can then be picked up by predators, such as seagulls. Min-till practices, which involve reduced cultivation of the soil, are believed to help soil regenerate, but have reduced exposure of wireworms to predation, thereby increasing their prevalence
Cover crops are plants that are planted to cover the soil rather than for the purpose of being harvested. They are used for preventing soil erosion, to increase the organic matter in the soil along with nutrients and nitrogen, for weed control and, crucially, in pest management [Sullivan, 2003]. When planted with a food crop, or in the season prior to the food crop, cover crops can provide an alternative food source for the wireworms and can also attract predators for biological control. Some plants, such as mustard and cabbage, contain glucosinolates that when hydrolysed form biologically active compounds that have provided effective control of soil-borne pests [Parker and Howard, 2001].
However, it is not all good news, since some cover crops end up being beneficial to the wireworms since they represent another food source, and those plants can help keep soil temperatures up particularly if they are a winter cover crop.
Wireworm populations can also be influenced by cropping history and crop rotation.
- Mustard and cabbage are rich in glucosinolate and rotation to these crops has been found to reduce soil wireworm populations [Lichtenstein et al., 1964] whilst using brassica meal in potato pots also reduces wireworm damage.
- In Idaho, a four to five year rotation with alfalfa has been reported to reduce wireworm populations in heavily infested fields [Shirck, 1945].
- Carrots and field beans have been shown to work well as catch crops in rotations with potato, where infestation levels were found to be significantly lower than in rotations which used undersown crops [SchepI and Paffrath, 2003].
- Buckwheat, Fagopyrum esculentum (Polygonaceae), has been reported to also decrease wireworm population levels in Nova Scotian potato and carrot fields when planted as cover or rotation crops, as it causes the pest to stop growing, leading to deformities and death [MacKenzie et al, 2010].
Attract and kill methods are a hybrid form of integrated pest management that combines pheromonal attraction with insecticides. Using wheat seed treated with fipronil and/or thiamethoxam reduced blemishes by 81.2 % [Vernon et al 2015] whilst CO2-releasing beads with a neem seed kernel extract within an attract-and-kill co-formulation can be used for the control of soil-dwelling larvae of herbivorous insect pests, in particular wireworms [Humbert et al, 2017]. Attract and kill strategies use minute amounts of insecticide/ha, have low environmental risk, and can be implemented at low cost with minor modifications to planting machinery.
Finally, there is some evidence that wireworm pressure might be reduced within complex landscapes [Basedow 1990; Ostman et al, 2001; Thies and Tscharntke 1999; Thies et al, 2003] and increased diversification is in line with much current thinking about sustainability of agricultural land. Adopting a more diverse rotation system, for example, can improve crop productivity and deliver enhance local ecosystems. It may also enhance environmental and economic benefits as it reduces yield loss without the negative consequences of pesticide.
Landscapes composed of arable fields interspersed with non-crop habitats, like woods and field margins, support higher biodiversity than landscapes consisting of only arable fields [Wrbka et al, 2008]. These areas will encourage the presence of predators, like birds and nematodes, which will help prevent imbalance between the predator and pest populations [HDRA, 1998]. Though grass margins can promote wireworm populations, they should still be integrated into the landscape in a way that is spatially and temporally more favourable to natural enemies than to pests through adequate management regimes [Hermann et al, 2012].
Conclusions
Our understanding of both wireworm and click beetle biology, as well as knowledge of the prevalence of both larval and adult forms, has witnessed significant advancements in recent years, but many challenges persist and are arguably getting worse rather than better. Regenerative agricultural approaches coupled with loss of key chemical controls have allowed wireworms to thrive and this threatens various sectors of the agricultural industry.
It is more important than ever that we continue with innovative research and aim towards integrated pest management strategies that will help to develop more sustainable and effective control measures. Finding new insecticides with reduced environmental impact and targeted application methods will be key to minimizing wireworm populations.
However, the complex life cycle and adaptability of wireworms continue to pose challenges. Continued collaboration between researchers, farmers, policymakers and business is crucial for furthering our understanding of wireworm behaviour and developing comprehensive control strategies. By combining technological advancements, sustainable practices, and knowledge sharing, we can move towards mitigating the damage caused by wireworms and ensuring the long-term health and productivity of agricultural systems
The author
Rebekah Neill is B-hive’s in house expert on wireworm. She has built a wealth of knowledge on these devastating pests and how they can be controlled. Rebekah has recently managed a highly successful feasibility project, funding by Innovate UK, to investigate how wireworms can be detected using computer vision-based methodologies.
References and further reading
Adhikari, A., and Reddy, G.V. (2017). Journal of Arthropod-Plant Interactions 11, 755-766.
Apablaza, J.U., A.J. Keaster, and R. H. Ward (1977). Environmental Entomology 6, 715-718.
Ansari, M., Evans, M., and Butt, T. (2009). Journal of Crop protection 28, 269-272.
Balachowsky, A., Mesnil, L. (1936). Lechevalier, P. ed.; Institut Agronomique de l’Etat. Chaire de Zoologie. Gembloux: Paris; Volume 2, p. 506
Barsics, F., Haubruge E., and Verheggen F.J. (2013) Insects 4, 117-152.
Basedow, T. (1990). Gesunde Pflanzen 42, 241–245.
BASF (2021). Cimegra. Available from https://agriculture.basf.ca/horticulture/en/products/cimegra.html [accessed 22nd November 2021].
Bechinski, E.J., Sandvol, L.E., Carpenter, G.P. and Homan, H.W (1994). Univ Idaho Coop Ext Circ, 760.
Blackshaw, R.P., and Vernon, R.S. (2006 Journal of Applied Ecology 43(4), 680-689.
Blackshaw, R.P., Hicks, H., and Vernon, R.S. (2008). Communications in Agricultural and Applied Biological Sciences 73(3), 429-432.
Boiteau, G., Bousquet, Y., and Osborn, W. (2000). Environmental Entomology 29(6), 1157-1163.
Brian, M.V. (1947). Journal of Animal Ecology 16(2), 210-224.
Burnell, A.M., and Stock, S.P. (2000). Journal of Nematology 2, 31-42.
Campbell, R.E. (1937). Ecology 18, 479–489.
Candide (2021). Wireworm. Available from https://candidegardening.com/US/insects/4a33aa4448ace3bdde9d0a3b950906b5
CBC (2021). Available from https://www.cbc.ca/news/canada/prince-edward-island/pei-wireworm-behaviour-research-1.6069053 [accessed 2nd December 2021].
CBC (2021Available from https://www.cbc.ca/news/canada/prince-edward-island/pei-new-pesticide-wireworm-potatoes-1.5935366 [accessed 23rd Feburary 2023].
Falconer, D.S., (1944). Journal of Experimental Biology 21, 17-32.
FAO (2005) FAOSTAT. Agriculture. Available at http://faostat.fao.org/faostat/collections?/subset=agriculture
Furlan L.,Contiero B.,Chiarini F., Colauzzi M., Sartori E., Benvegnù I., Fracasso F. and Giandon P. (2017) Environ Sci Pollut Res Int. 24(1): 236–251.
Grove, I.G., Woods, S.R., and Haydock, P.P.J. (2000). Annals of Applied Biology 137, 1-6.
Jansson, R.K., and Seal, D. (1994). Advances in Potato Pest Biology Management. APS Press, 31-53.
HDRA (1998). Natural Pest and Disease Control (online). Available at https://www.gardenorganic.org.uk/sites/www.gardenorganic.org.uk/files/resources/international/PestDisease.pdf [accessed 2nd December 2021].
Hermann, A., Brunner, N., Hann, P., Wrbka, T., and Kromp, B. (2012). Journal of Pest Science 86, 41–51.
Humbert, P., Vemmer, M., Mavers, F., Schumann, M., Vidal, S., and Patel, A.V. (2017). Pest Management Science 74(7), 1575-1585.
Irish Farmers Journal (2019). Potato growers to lose key active for battling wireworms [online]. Available at https://www.farmersjournal.ie/potato-growers-to-loose-key-active-for-battling-wireworms-444980 [accessed 3rd December 2021].
Jaronski, S.T. (2010). Biocontrol 55, 159–185.
King R., Buer B., Davies T.G.E., Ganko E., Guest M., Hassani-Pak K., Hughes D., Raming K., Rawlings C., Williamson M., Crossthwaite A., Nauen R. and Field L. (2023) Pesticide Biochemistry and Physiology, Volume 191, April 2023, 105339
Kozina, A., Čačija, M., Barčić, J.I., and Bažok, R. (2012). International Journal of Biometeorology 57 (4), 509-519.
Kozina, A., Lemic, D., Bazok, R., Mikac, K.M., Mclean, C.M., Ivezić, M., and Barčić, J.I. (2015). Journal of Insect Science 15(1).
Kuhar, T.P., Alvarez, J.M. (2008) Crop Protection 27, 792-798.
Ladurner E, Benuzzi M, Fiorentini F & Franceschini S (2008) In Proceeding of Ecofruit-13th International Conference on Cultivation Technique and Phytopathological Problems in Organic Fruit-Growing, 93-97.
La Forgia, D., Thibord, J.B., Larroude, P., Francis, F., Lognay, G., Verheggen, F. (2019). Journal of Pest Science 93, 605-614.
Lefko, S.A., Pedigo, L.P., Batchelor, W.D., and Rice, M.E. (1998) Environmental Entomology 27(2), 184-190.
Lewis, E.E., Campbell, J., Griffin, C., Kaya, H., and Peters, A. (2006). Journal of Biological control 38, 66-79.
Lichtenstein E, Morgan D & Mueller CFC (1964) Journal of Agricultural and Food Chemistry 12, 158-161.
MacKenzie, J., Nelson, J., and Hammermeister, A. (2010). Management practices for control of European wireworms in Canada. Interim Research Report E2010-37. Organic Agriculture Center of Canada. Truro, Nova Scotia.
Milosavljevica I., Esserb A.D. and Crowder D.W. (2015) Agriculture, Ecosystems and Environment 225 (2016) 192–198
Nikoukar, A., and A., Rashed (2022). Insects, 13 (769), 1-24.
Ostman, O., Ekbom, B., Bengtsson, J. (2001). Basic and Applied Ecology 2, 365–371.
Parker, W.E., and Howard, J.J. (2001) Agricultural and Forest Entomology 3(2), 85-98.
Parker W.E. (1994) Crop Protection 15(6), Pages 521-527
Parker, W.E., and Seeney, F.M. (1997). Annals of Applied Biology 130, 409-425.
Purdue University (2009). Wireworms. Available from https://extension.entm.purdue.edu/fieldcropsipm/insects/corn-wireworms.php [accessed 17th Feb 2023].
Poggi, S., Le Cointe, R., Lehmhus, J., Plantegenest, M., and Furlan, L. (2021) Agriculture 11, 1-30.
Poggi, S., Le Cointe, R., Riou, J.B., Larroude, P., Thibord, J.B., and Plantegenest, M. (2018). Journal of Pest Science 91, 585–599.
Rashed, A., Etzler, F., Rogers, C., and Marshall, J. (2015). J University of Idaho Extension Bulletin 898.
Reinbacher, L., Bacher, S., Knecht, F., Schweizer, C., Sostizzo, T., and Grabenweger, G. (2021). Crop Protection 150, 1-10.
Ritter, C., and Richter, E. (2013). Journal of Plant Diseases and Protection 120(1), 4-15.
Roebuck, A., Broadbent, L., and Redman, R.F.W. (1947). Ann Applied Biology 34, 186–196.
Rogge, S.A., Mayerhofer, J. Enkerli, J., Bacher, S., and Grabenweger, G. (2017). BioControl 62, 613–623.
Salt, G., and Hollick, F.S.J. (1946). Journal of Experimental Biology 23, 1-46.
Salt, G., and Hollick, F.S.J. (1949). Annals of Applied Biology 36 (2), 169-186.
Saussure S., Plantegenest M., Thibord J.-B., Larroudé P. & Poggi S. (2015) Agronomy for Sustainable Development volume 35, pages793–802 (2015)
Schallart, N., Tusch, M.J., Staudacher, K., Wallinger, C., and Traugott, M. (2011). Soil Biology and Biochemistry 43, 1612-1614.
Schepl. U, & Paffrath, A, (2003) Scientific Conference on Organic Agriculture. Vienna: University of Agricultural Sciences, Institute for organic farming., pp 133-136. Available at https://www.semanticscholar.org/paper/The-Development-of-Strategies-to-Regulate-the-of-in-Schepl-Paffrath/864835c4a54a48245705c50a36e6b7712592b973 [accessed 2nd December 2021].
Shirck FH (1945) Journal of Economic Entomology 38, 627-633.
Smith, D.B., A.J. Keaster, J.M. Cheshire, and R.H. Ward (1981). Journal of Economic Entomology 74, 625-629.
Staudacher, K., Pitterl, P., Furlan, L., Cate, P.C. and Traugott, M. (2011). Bulletin of Entomological Research , Volume 101 , Issue 2 , April 2011 , pp. 201 – 210 DOI: https://doi.org/10.1017/S0007485310000337
Sufyan. M., Abbasi, A., Gogi, M.D., Arshad, M., Nawaz, A., and Neuhoff, D. (2017). J Gesunde Pflanzen 69, 197-202.
Sufyan, M., Neuhoff, D., and Furlan, L. (2007). Integrated Control of Soil Insect Pests 30(7), 83-87.
Sufyan, M., Neuhoff, D., and Furlan, L. (2014). Bulletin of Insectology 67 (2), 227-235.
Sullivan, P. (2003) Overview of cover crops and green manures. Fundamentals of sustainable agriculture, ATTRA. Available at: https://cpb-us-e1.wpmucdn.com/blogs.cornell.edu/dist/e/4211/files/2014/04/Overview-of-Cover-Crops-and-Green-Manures-19wvmad.pdf [accessed 1st December 2021].
Thies, C., Steffan-Dewenter, I., and Tscharntke, T. (2003). Oikos 101, 18–25.
Thies, C., and Tscharntke, T. (1999). Science 285, 893–895.
Triplehorn, C., and Johnson, N. (2005). Borror and DeLong’s Introduction to the Study of Insects. J Brooks Cole. Belmont, CA.
UK Beetles (2021). https://www.ukbeetles.co.uk/agriotes-lineatus [accessed 25th September 2021].
UtahStateUniversity (2020). Wireworms. Available from https://extension.usu.edu/pests/research/wireworms [accessed 16th February 2023].
van Herk, W., and Vernon, R.S. (2020). Environmental Entomology 49(2), 449–460.
van Herk, W., Vernon, R.S., Clodius, M., Harding, C., and Tolman, J.H. (2007). Journal of the Entomological Society of British Columbia 104, 55-64.
Van Herk, W.G., Vernon, R.S., Richardson, J., Richardson, M., and Beaton, A. (2021). Florida Entomologist 104(1), 42-50.
Vernon, R.S., and van Herk, W., (2013). Insect Pests of Potatoes. Elsevier Inc: 103-164.
Vernon, R.S., van Herk, W.G., Clodius, M., and Tolman, J. (2015). Journal of Pest Science 89(2), 375-389.
Vernon, R.S., van Herk, W.G., Tolman J., Ortiz Saavedra, H., Clodius, M., and Gage, B. (2007). Journal of Economic Entomology 101(2), 365-374.
Washington State University. Wireworms. Available from https://smallgrains.wsu.edu/insect-resources/pest-insects/wireworms/ [accessed 24th February 2023].
Wrbka, T., Schindler, S., Pollheimer, M., Schmitzberger, I., and Perterseil, J. (2008). Community Ecology 9, 217–227.
Yonuis, M.I., Abdulhay, H.S., Hameed, A.A. (2015). World Journal of Pharmaceutical Research 5(1), 1390-1407.